Ether synthesis
Williamson Ether Synthesis
Ethers are often synthesized from alcohols. One alcohol is turned into an alkyl halide or a tosylate ester. With this good leaving group, it can be attacked by an alkoxide made from an alcohol and sodium. This SN2 reaction produces an ether. This is called the Williamson Ether Synthesis.
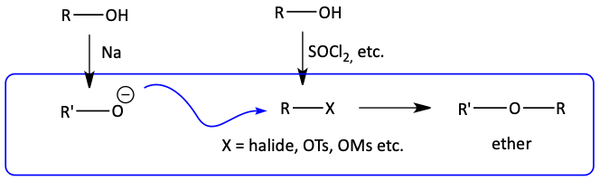
Williamson Ether Synthesis from Alcohols
Alkoxymercuration and Demercuration in alcohol
Mercuric acetate can react with an alkene to make the triangular mercurium ion. This is an example or a triangle reaction. If water is used as the solvent, a Markovnikov alcohol is formed. If water is not used as the solvent, it cannot react as the nucleophile. If alcohol is used as the solvent, the lone pair of electrons on the alcohol can attack the mercurium ion as a weak nucleophile. Again, it attacks carbon atom of the mercurium triangle with the greatest partially positive charge. The electron bond goes up onto the mercury cation to make a good, neutral mercury leaving group. The protonated –OR group is deprotonated with another molecule of solvent to make a mercury-ether containing compound. Many different Markovnikov ethers can be made depending on the alcohol used as the solvent. R = CH3 for methanol, R = CH2CH3 for ethanol, etc.
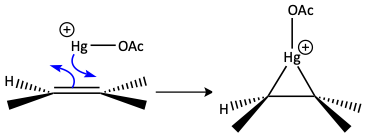
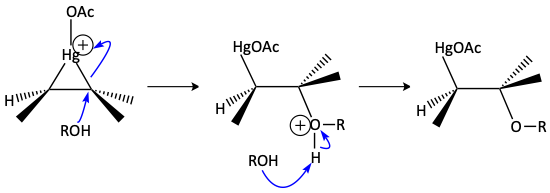
Sodium borohydride (NaBH4) is used to replace HgOAc with H- making a Markovnikov ether.
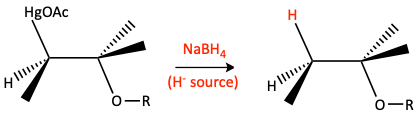
Bimolecular dehydration
We saw in the last chapter that an industrial way to synthesize ethers is through the bimolecular dehydration of alcohols. In the presence of a dehydrating acid, like sulfuric (H2SO4), phosphoric (H3PO4), the hydroxyl groups can be protonated making good water leaving groups. This way to make ethers is much less common in an organic chemistry lab because the reaction conditions are fairly harsh and since carbocations are formed, rearrangements often happen.

General reaction for bimolecular dehydration
It is also necessary to use two of the same alcohols to make symmetrical ethers. Otherwise, a mixture of several ethers is formed.

The mechanism for this ether-forming dehydration involved protonating a hydroxyl group of an alcohol to make the great, stable, H2O leaving group. The other alcohol attacks via an SN2, backside-attack, mechanism. After cleaning it up, an ether is formed. Below, see the mechanism for the dehydration of two molecules of ethanol to form diethyl ether. This dehydration to form an ether tends to happen around 130-140°C.
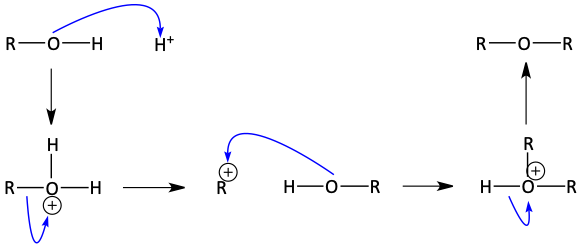
Mechanism for acid catalyzed bimolecular dehydration to make ethers
As seen in the previous chapter, a competing reaction to the bimolecular dehydration of two alcohols to form an ether is the unimolecular dehydration of an alcohol to form an alkene. This tends to happen when the temperature gets over 140°C. This dehydration can occur with sulfuric acid, H2SO4, phosphoric acid, H3PO4, and p-toluenesulfonic acid (tosic acid, p-TsOH).
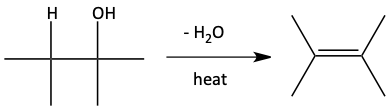
2. Give two distinct syntheses of ethyl isopropyl ether. Both syntheses must use ethanol as one of the starting materials.
Sulfide Synthesis
Sulfides, or thioethers, are the sulfur analogues of ethers. They can be synthesized by using a reaction similar to the Williamson Ether synthesis. Thiols, the sulfur analogue of alcohols, can be deprotonated making nucleophilic thiolate anions. The thiolate anions can then perform an SN2 reaction on an alkyl halide resulting in a sulfide or thioether.
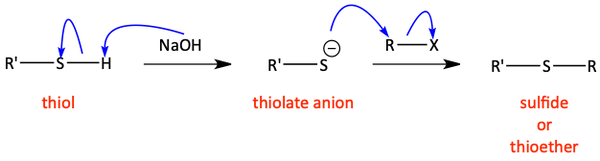
Remember that sulfur is directly below oxygen in the periodic table. Therefore, sulfur atoms are larger than oxygen atoms. Thiols are easier to deprotonate than alcohols, because the negative charge produced in a thiolate anion is spread out over a larger sulfur atom compared to the negative charge on an oxygen atom of an oxide. A base like sodium hydroxide can be used to deprotonate the thiol. The deprotonation of alcohols took a stronger base, like sodium or sodium hydride.
Thiolate anions are also very good nucleophiles because the negative sulfur atom is large and polarizable. The fluffy electron cloud of the thiolate anion can easily reach out its electrons to start the SN2 attack of the alky halide. They are such good nucleophiles; they will even attack secondary alkyl halides.
Answers
2.
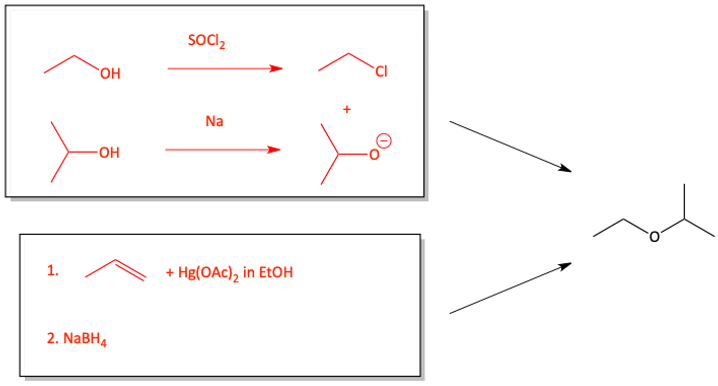